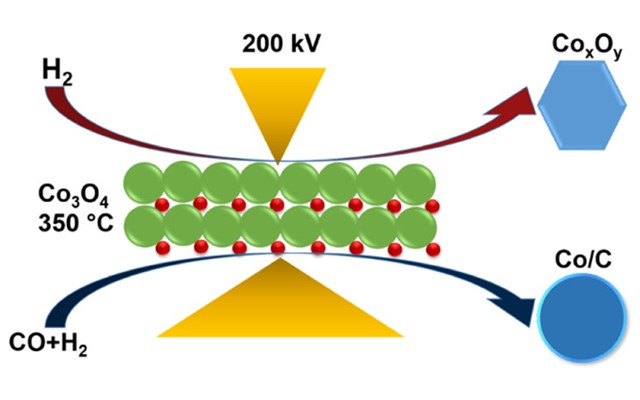
Hydrogen vs. syngas: The battle of the reducing gases
Using our Climate system, scientists are able to unveil the most effective method to obtain sintering-resistant metallic cobalt nanoparticles at lower temperatures.
Original article by Ofentse A. Makgae, Tumelo N. Phaahlamohlaka, Benzhen Yao, Manfred E. Schuster, Thomas J. A. Slater, Peter P. Edwards, Neil J. Coville, Emanuela Liberti, and Angus I. Kirkland
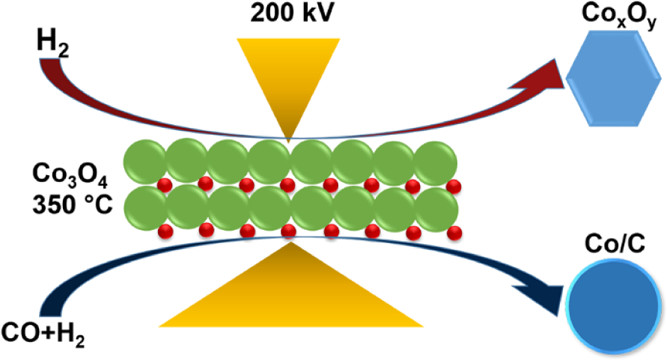
The Fischer-Tropsch synthesis (FTS) is an established catalytic chemical process that occurs when carbon monoxide and hydrogen are converted into hydrocarbons. These hydrocarbons can be further developed into high-quality fuels, lubricants as well as raw materials for the production of plastics, rubbers and industrial chemicals. In FTS, supported cobalt catalysts are commonly used, which are active in their reduced metallic state. Many factors can affect the reduction process of cobalt oxide (Co₃3O₄) to metallic cobalt, including the reduction temperature, the composition of the reducing gas, the catalyst promoter, catalyst support and particle size. Despite this, little is known about the effect of using H₂ versus syngas (H₂ & CO) as the reducing agent on carbon-supported cobalt catalysts.
In recent research performed at the David Cockayne Centre for Electron Microscopy and the electron Physical Science Imaging Centre (ePSIC), a number of scientists from highly renowned universities and institutes, including University of Oxford, Johnson Matthey, Diamond Light Source and Cardiff University, investigate the atomic structure and valence state of cobalt nanocrystals on carbon supports under syngas versus hydrogen reduction. Specifically, using ex situ and in situ high-resolution aberration-corrected analytical electron microscopy with our Climate system, the researchers were able to explore the effect of H₂ versus syngas (H₂ & CO) on the reducibility of cobalt oxide nanoparticles supported on hollow carbon spheres. By uncovering highly effective methods to obtain sintering-resistant metallic Co nanoparticles at lower temperatures on hollow carbon sphere supports, this work provides valuable insights that can inform important industrial processes.
Synthesis and reduction of cobalt oxide
Dr. Ofenste Makgae and his fellow collaborators first synthesized the Co₃3O₄ nanoparticles before preparing the hollow carbon sphere supports (HCSs). The synthesized Co₃3O₄ nanoparticles were then loaded on the surface of the HCSs to prepare the precursor catalyst.
The researchers then initiated the reduction process of the Co₃3O₄ nanoparticles for both the ex situ and in situ analysis. For the ex situ analysis, the precursor was reduced via H₂ and syngas, respectively, at 350 °C at atmospheric pressure for 20 hours. For the in situ analysis, the catalysts were studied using the DENSsolutions Climate system in a probe-corrected JEOL ARM200F at ePSIC operated at 200 kV. Notably, the DENSsolutions Climate system is capable of real-time dynamic mixing of 3 gases over a temperature range from room temperature to 1000 °C. Via the Climate Gas Supply System, Dr. Makgae and his fellow collaborators were able to provide a 0.2mL/min flow of gas at a pressure of 500mbar for both the H₂ and syngas reduction. The temperature was ramped from room temperature to 150 °C under a N₂ flow to remove contaminants. Next, it was ramped to 350 °C at 10 °C/min under a reducing environment. Finally, the temperature was held at 350 °C for 2 hours for catalyst activation.
Ex situ post-reduction morphology analysis
The researchers then set out to analyze the overall morphology of the carbon support post reduction, to ensure that its integrity was not compromised during the reduction process. In Figure 1 below, (high-resolution) STEM-ADF images of the as-prepared Co₃3O₄ and ex situ syngas- and H₂-reduced HCS-supported nanoparticles are shown. The researchers were able to determine that the morphology of the HCSs remained intact after 20 hours of reduction at 350 °C.
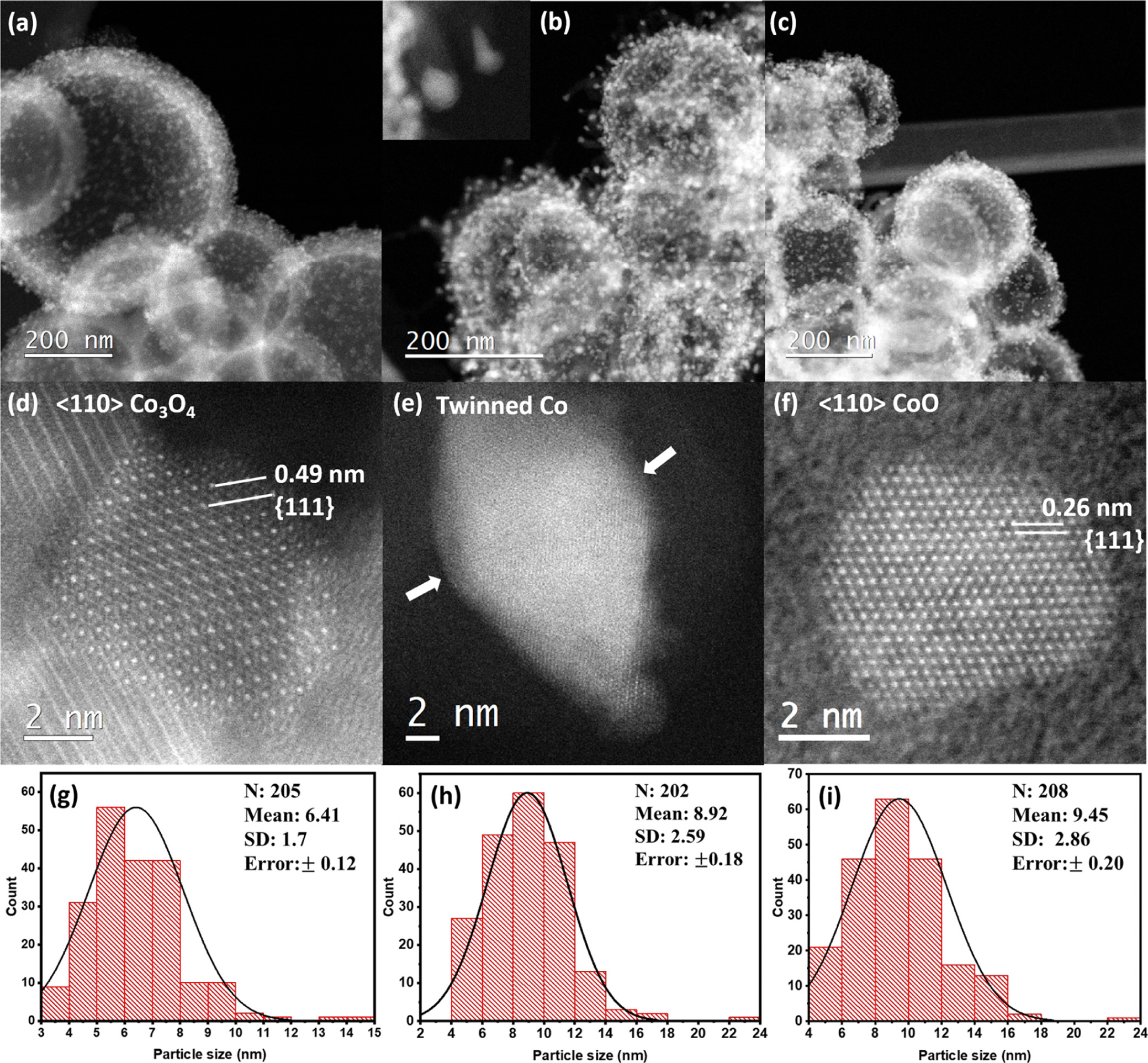
Figure 1: STEM–ADF images of the Co nanoparticles (a) before and after the ex situ reduction in (b) syngas (H₂/CO = 2) and (c) H₂ at 350 °C. (d–f) are the corresponding high-resolution STEM–ADF images of the as-prepared Co₃3O₄, syngas-, and H₂-reduced nanoparticles.
EELS spectra and elemental maps
Aside from morphological changes, the oxidation state of cobalt also changes during the reduction process as a function of the reducing gas composition. Using STEM–EELS, the researchers were able to thoroughly investigate the Co-oxidation state changes. Figure 2 below compares the EELS spectra and elemental maps of single particles of the as-prepared Co₃3O₄, and the syngas-reduced and H₂-reduced nanoparticles. Via the EELS spectra analysis, the researchers determined that there was indeed a significant decrease in the oxidation state of Co.
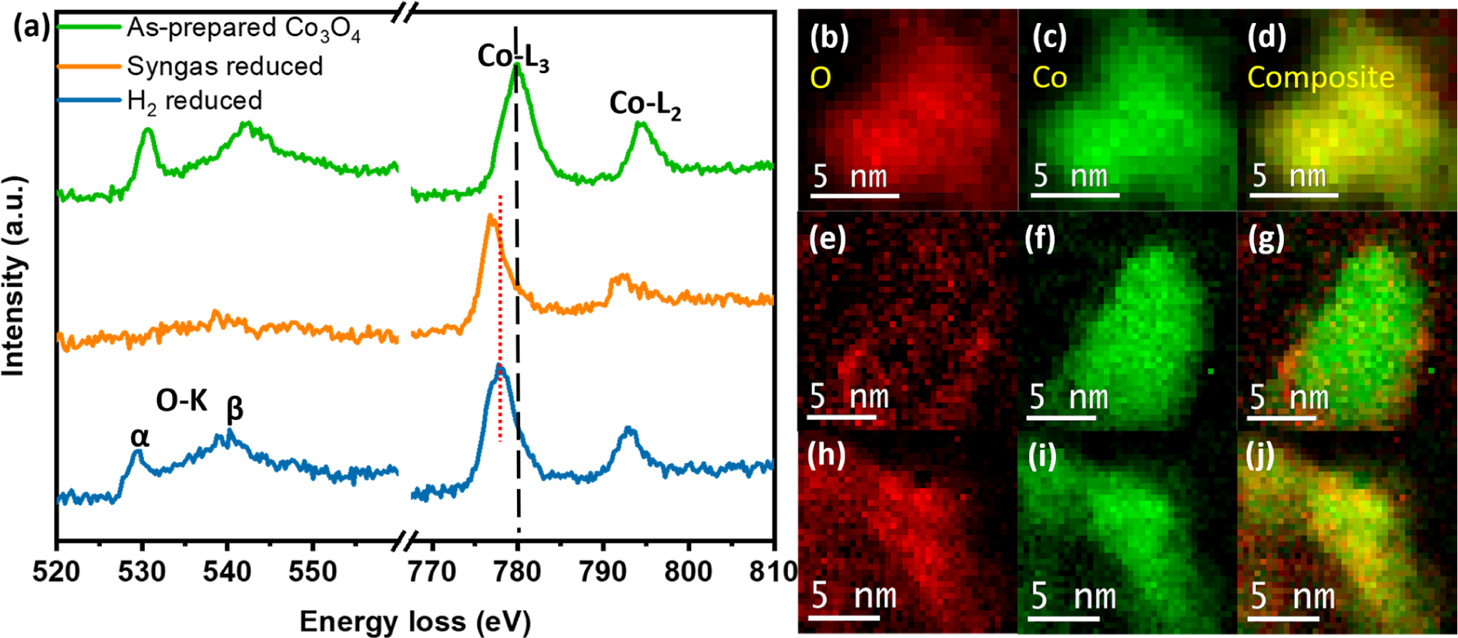
Figure 2: EELS spectra of the as-prepared Co₃3O₄ (green), syngas- (orange), and H₂- (blue) ex situ reduced nanoparticles at 350 °C. EELS elemental maps of (b–d) as-prepared, (e–g) syngas-reduced, and (h–j) H₂-reduced Co₃3O₄ nanoparticles.
Importantly, the EELS spectra observations also show that the degree of reduction varies amongst the two methods (H₂ and syngas). To explore this further, the researchers collected additional spectra to compare the ex situ syngas-reduced and H₂-reduced nanoparticles at 350 °C, with the as-prepared Co₃3O₄ as a reference. From this further analysis, it is observed that the Co in the case of the syngas-reduced nanoparticles’ spectra is in a near-metallic state as compared to the H₂-reduced nanoparticles. These results are consistent with the elemental maps obtained, showing that a more intense Co signal (green) is observed in the elemental map from a syngas-reduced nanoparticle (Figure 2g), compared to a more diffuse Co signal in the elemental map of a H₂-reduced nanoparticle (Figure 2j). Ultimately, in the absence of air surface reoxidation, the results of the in situ STEM–EELS and ADF analysis show that syngas achieves a higher degree of Co reduction than H₂.
High-resolution STEM-ADF analysis
The next step for Dr. Makgae and his collaborators was to perform high-resolution STEM–ADF imaging in order to investigate the differences in the atomic structure associated with the observed differences in Co-oxidation states in the syngas- and H₂-reduced nanoparticles. They explored the reduction of the syngas- and H₂-reduced nanoparticles at two different temperatures, 350 °C and 600 °C.
1) At 350 °C
In Figure 3a–e below, the high-resolution STEM–ADF images of syngas-reduced nanoparticles (a–c) and H₂-reduced nanoparticles (d,e) are presented. The results were found to be consistent with the EELS data, showing that syngas reduction produces a majority of metallic Co nanoparticles, whereas H₂ produced particles with a majority of the Co in the 2+ oxidation state.
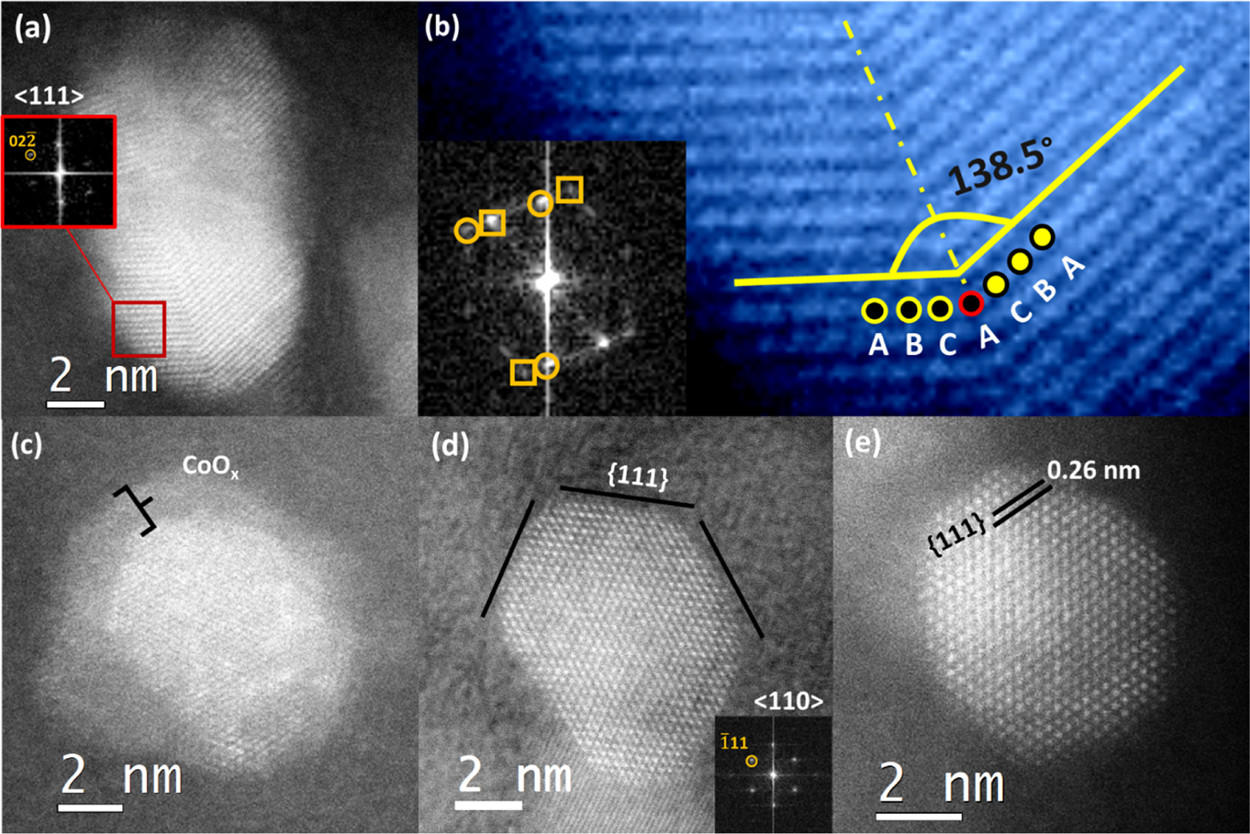
Figure 3: High-resolution STEM–ADF images of ex situ (a–c) 350 °C syngas-reduced nanoparticles and (d,e) H₂-reduced nanoparticles.
2) At 600 °C
The temperature was then increased to 600 °C to investigate the effect of temperature on reducing HCS-supported Co₃3O₄ nanoparticles under H₂ versus syngas. It is observed that both the H₂- and syngas-reduced nanoparticles exhibit an atomic structure consistent with cobalt (Figure 4a–d). Indeed, the results are consistent with the EELS data from the 600 °C reduced nanoparticles, which shows the presence of metallic cobalt. Conclusively, in order to achieve a complete reduction of Co₃3O₄ under H₂, the HCS-supported nanoparticles must be reduced at high temperatures. Importantly, it is observed that the high temperature of 600 °C resulted in severe sintering of the nanoparticles reduced in H₂ compared to syngas. The sinter-resistance in syngas-reduced nanoparticles is attributed to the encapsulation of nanoparticles with a carbon shell (indicated by the orange arrow in Figure 4b). This encapsulation prevents the coalescence of the nanoparticles at high temperatures. Interestingly, in the case of the H₂-reduced nanoparticles, a carbon shell is not observed (Figure 4d).
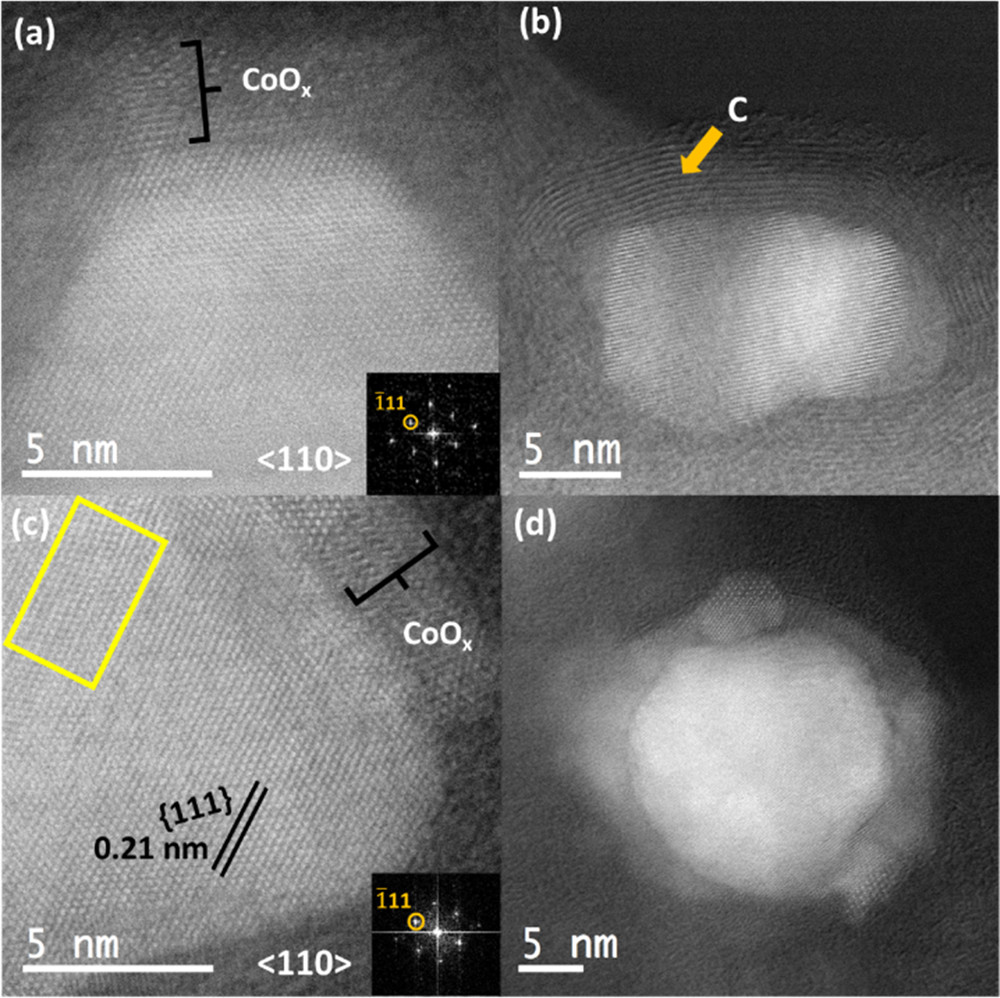
Figure 4: High-resolution STEM–ADF images of 600 °C ex situ (a,b) syngas-reduced nanoparticles and (c,d) H₂-reduced Co nanoparticles.
Conclusively, the presence of CO in syngas is observed to play a critical role in the conversion of Co₃3O₄ to metallic Co at 350 °C. In contrast, the full reduction of Co₃3O₄ to active metallic Co in H₂ is not achieved at 350 °C. Increasing the activation temperature to 600 °C does drive the complete reduction, however, significant particle sintering was still observed. Therefore, the presence of CO in a syngas mixture is indeed crucial to achieving complete Co₃3O₄ reduction at 350 °C.
Conclusion
This paper comprehensively illuminates the most effective method to obtain sintering-resistant metallic cobalt nanoparticles at lower temperatures under carbon supports. By conducting a thorough ex situ and in situ electron microscopy analysis using the DENSsolutions Climate system, Dr. Makgae and his fellow collaborators were able to observe under nanometer resolution how the reduction process under H₂ versus syngas affects the physicochemical properties of the reduced nanoparticles. The findings show that syngas achieves a higher reduction at an industrially relevant Fischer-Tropsch reduction temperature of 350 °C compared to H₂ on carbon supports. Importantly, lower reduction temperatures are more efficient as operating costs are reduced, which is a significant consideration for organizations involved in industrial processes. The discovery of such findings could not be possible without the application of in situ electron microscopy techniques, for which our Climate system has proved to be remarkably valuable.
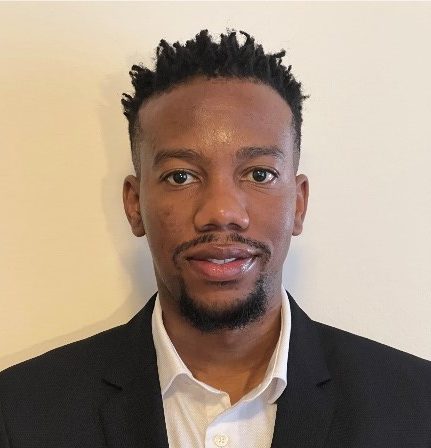
“The DENSsolutions Climate system allowed us to directly evaluate the reducibility of Fischer-Tropsch catalysts under industrially relevant temperatures. Such observations provide a unique insight into the evolution of catalysts under activation conditions.
Dr. Ofentse Makgae
Post-doctoral Research Fellow | Lund University
Original article:
Discover our Climate solution:
Discover more publications made possible by Climate:
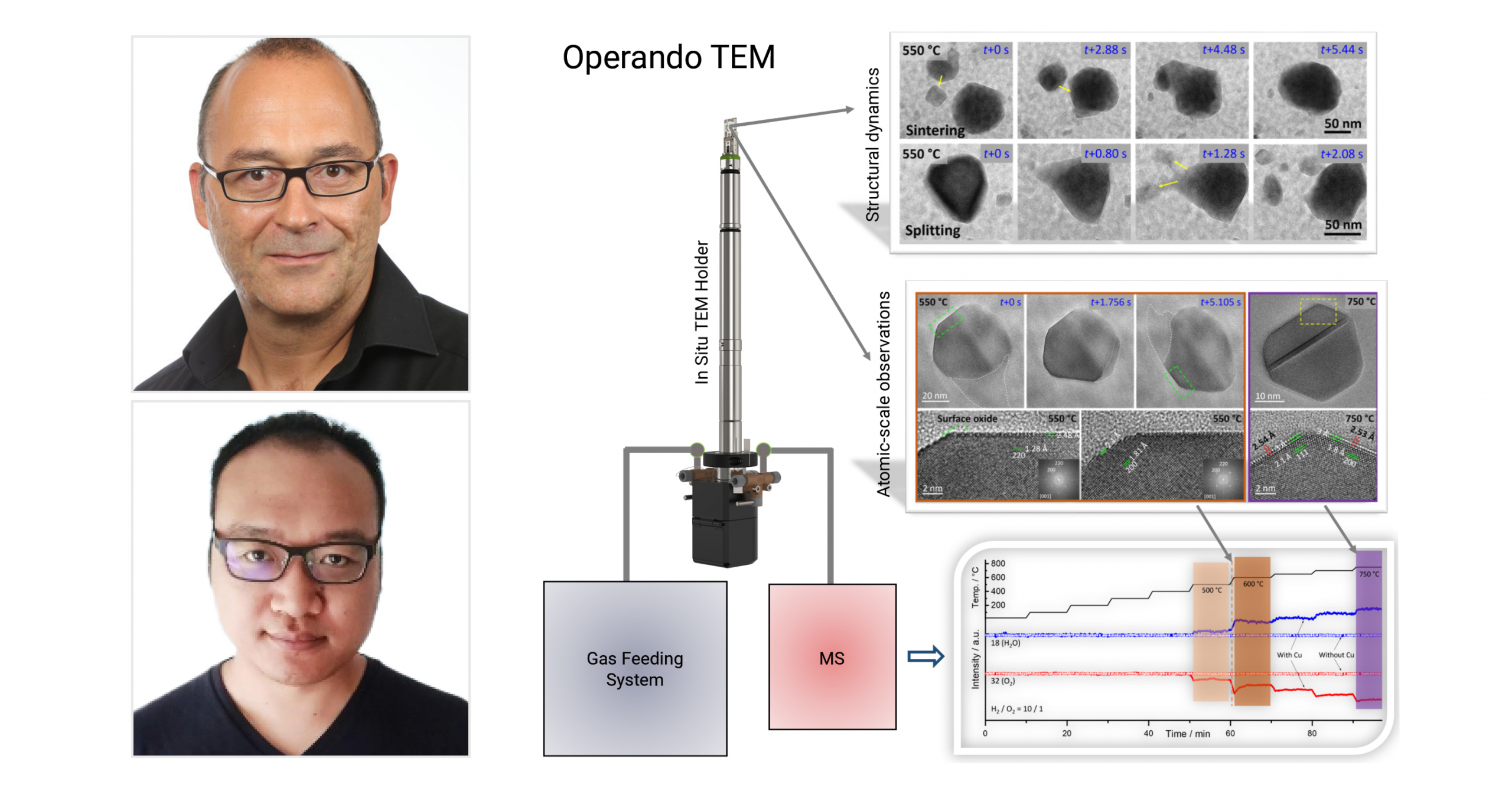
Climate helps uncover phase coexistence and structural dynamics of redox metal catalysts
Using our Climate system, scientists are able to interrelate the atomic-scale structural dynamics of redox metal catalysts to their activity.
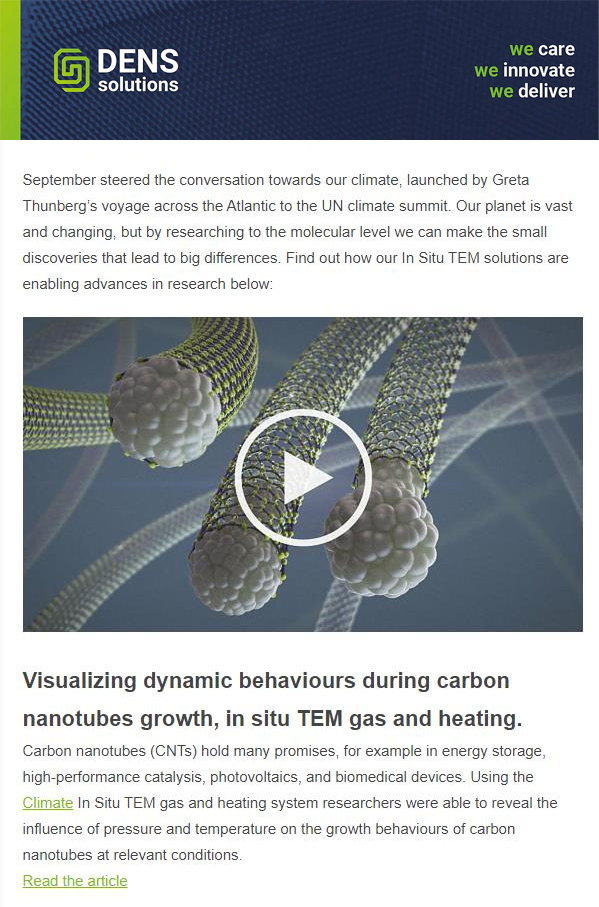